Abstract
This article is compiled from a report conducted by nova-Institute and commissioned by Unilever. In this report the total global amount of embedded carbon in products from the chemical and derived material sector is examined.
It shows how this demand is met today and recommends the strategies to meet the embedded carbon needs by 2050 in the transition from fossil to renewable carbon sources. In this report, a comprehensive policy framework for carbon management is discussed, which is necessary to realise the revolutionary transformation of the chemical industry, within a timeline that is in accordance with our climate targets.
Edited excerpts of a few important aspects from the report “Turning Off the Tap for Fossil Carbon” are presented here.
The full report can be downloaded for free:
www.renewable-carbon.eu/publications/product/turning-off-the-tap-forfossil-carbon-future-prospects-for-a-global-chemical-and-derived-material-sector-based-on-renewable-carbon/
Introduction
The climate crisis is accelerating at an unprecedented rate, with global warming, greenhouse gas emissions and deforestation causing food insecurity, global health concerns and biodiversity loss.
Greenhouse gas emissions linked to the use of fossil- based energy sources such as oil, coal and natural gas are the main factors contributing to climate change. It has become evident that we can no longer ignore the consequences that the current production methods we have on our planet. Increasing pressure from governments, investors and other stakeholders to transition to renewable energy sources has accelerated the development of solar power, wind farms and hydroelectricity, and of new technologies such as electromobility and green hydrogen. These innovations, however, are not being implemented fast enough. To decarbonise the energy sector by 2050, and ensure the achievement of the goals set out under the Paris agreement, it is essential for the industry to completely phase out its use of fossil fuels.
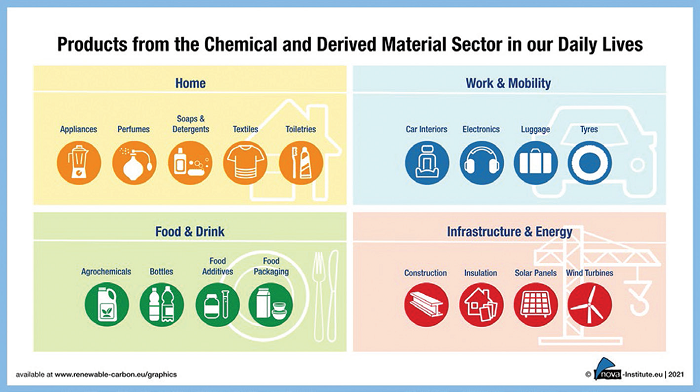
The chemical industry today relies almost entirely (85%) on virgin fossil carbon as a building block for its products. 92% of global warming impacts are caused by carbon-containing greenhouse gas emissions. Out of these, 80% are of fossil origin. In total, 89% of the carbon extracted from the ground is utilised for energy and fuels, whilst 11% is employed for cement, chemicals and derived materials. The latter will rise tremendously by 2050. The process of “decarbonising” the energy sector and expanding the use of renewable energies is ongoing in many countries.
Carbon Consumed in Chemical and Allied Products
Currently, the chemical sector consumes 14 percent of global oil and 8 percent of global natural gas supply, that represents 723 megatons (Mt) of pure carbon (equivalent to 2,655 Mt of CO2) 5. The largest share of the chemical manufacturing industry, be it plastics or detergents, is still fed by virgin fossil resources. However, decarbonising chemicals is not possible, as carbon is a key element of any chemical compounds. Therefore, the future share of the global oil and gas demand for carbon-based products will sharply increase.
Most of the carbon consumed by the chemical and derived material sector is made from fossil fuels – as is the case for the energy and fuel sectors. This poses a large issue, as the fossil carbon extracted from the ground (oil, gas and coal) eventually ends up in the atmosphere, contributing heavily to global warming. More than 50% of the extracted fossil carbon is released into the atmosphere as CO2 within a short amount of time; this includes plastic and rubber products that are incinerated, or detergents and cleaning agents that simply biodegrade. The remaining percentage initially remains in the technosphere in the form of products, and, depending on logistics and infrastructure, will later also be incinerated or biodegrade (in the environment and landfills) over decades to centuries. In the context of the circular economy, ever larger parts will be recycled in the future. Only then will the influx of emissions into the atmosphere end.
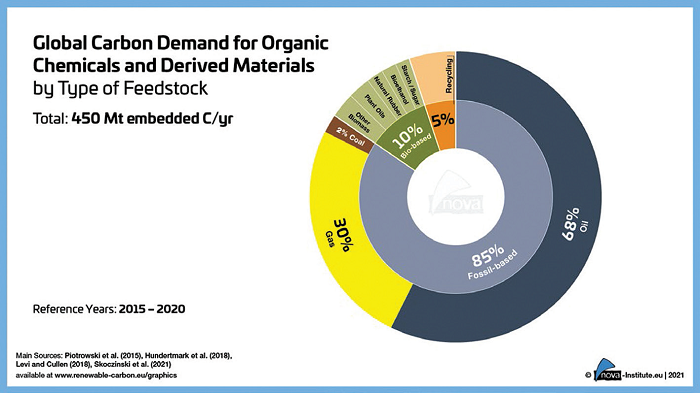
The demand for carbon embedded in organic chemicals and their derived materials is 450 million tons (Mt) per year. 85 percent of this demand is generated by fossil-fuel-based resources, 10% by biomass and only 5 percent by recycling. To create long-lasting and sustainable change, three sources of renewable carbon have been identified that can substitute the utilisation of fossil carbon that is extracted
from the ground: biomass, recycling, and CCU (Carbon Capture and Utilisation; captured CO2, from industrial processes or the atmosphere).
The demand for embedded carbon is set to rise. Increasing population, higher incomes and a growing middle class will drive the need for products and thus also for carbon. By 2050, nova-Institute estimates that the demand for carbon embedded in organic chemicals and derived materials will increase to 1,000 Mt per year. This would necessitate renewable carbon production to be increased by a factor of 15 by 2050. To achieve this demand sustainably, sharing, reusing and recycling will play the main role in keeping carbon in a closed loop, in line with the Circular Economy. Chemical and mechanical recycling industries will be largely responsible for innovating their processes to better reuse and recycle carbon. Since keeping the entire carbon in a cycle is technologically not possible, additional renewable carbon sources such as biomass and CO2 capture and use become necessary. For both options, sufficient land is available for either cultivation of biomass or the production of the required renewable energy for CCU. With these three renewable carbon sources (recycling, biomass and CCU) combined, it will be possible to keep using all the products we are used to without the need for any additional fossil carbon sourced from under the ground.
For the first time since the beginning of the industrial revolution, which was only possible due to the access to cheap fossil carbon sources, we are able to completely decouple the chemical and derived materials industries from virgin petrochemicals. Technologies, as well as investment capital, are available for the transformation from fossil to renewable carbon of the entire economy.
The renewable carbon family is the only pathway to a sustainable future for commonly used materials such as plastics, fibres, surfactants and other materials based on organic chemistry, and the industries that produce them. Adequate carbon management can aid companies in achieving their emission targets and allow them to resolve potential questions such as:
• In a given situation, what is the best choice from the renewable carbon family? Biomass, CO2 capture or recycling?
• Which renewable carbon source is the most sustainable, efficient and socially acceptable solution for a certain application in a given region?
• Is it biomass from wood, sugar beet or metropolitan biogenic waste?
• Is it captured CO2 from fossil power plants, from fermentation or from the atmosphere (direct air capture)?
• Or is it recycled carbon from old plastics via mechanical or chemical recycling?
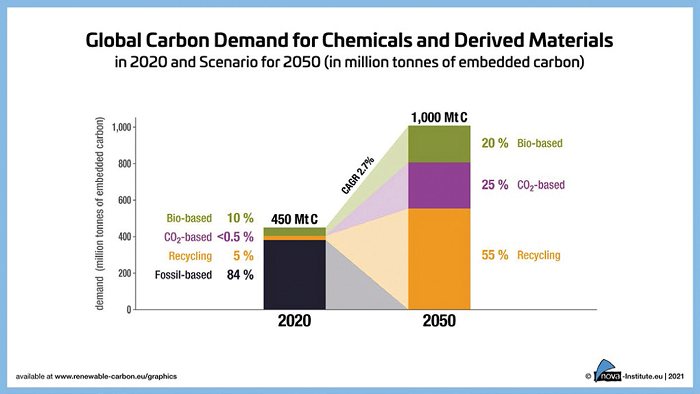
Once the need for transitioning the entire economy towards the use of renewable carbon is established, these are the questions to be addressed on a case-bycase basis taking into account the desired use and available infrastructure.
The exclusive use of renewable carbon as feedstock is a key condition for the chemical industry to achieve climate neutrality. The use of renewable carbon in the chemical and derived material industry is what decarbonisation is in the energy sector.
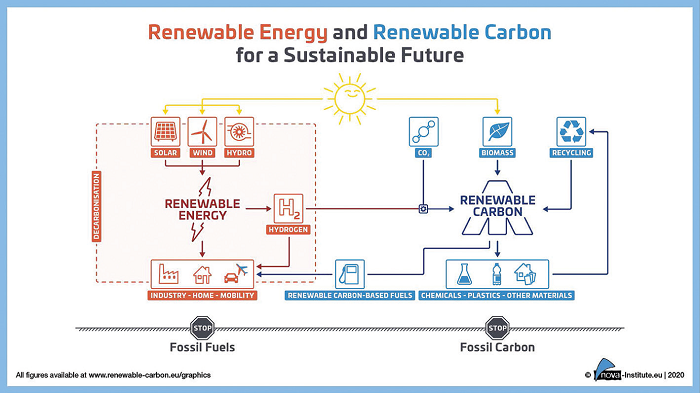
Fossil Carbon Strategies
Fossil carbon comes from oil, natural gas or coal, and eventually ends up in the atmosphere, contributing heavily to climate change. The largest challenge for the chemical industry is to convert its feedstocks from virgin fossil based to renewable-carbon-based.
The chemical industry is a key for a variety of other industries and products. It is a highly interconnected, integrated industry and has been optimised in many ways over decades. If this system is to be fundamentally changed, well considered strategies are required.
Comprehensive carbon management needs to take full account of the structure of today‘s chemical industry, preserve or repurpose industrial assets where possible, and replace those that have no future in a renewable carbon world.
There are therefore two different strategies for replacing virgin fossil-based products, both of which are important for the transformation and should be developed in parallel.
Strategy 1: Drop-in
The drop-in strategy uses existing structures of the chemical industry, such as refineries
and chemical parks, to initiate the raw material transformation at the feedstock level. Instead of naphtha, methane, ethane, propane methanol (see Figure 9) from fossil sources such as oil, natural gas and coal, the raw materials could be obtained from biomass, CO2 and chemical recycling. The end product stays the same, while the feedstock becomes renewable, and the existing processes and infrastructure largely remain in place. In this case, large amounts of virgin fossil carbon can be substituted quickly.
Strategy 2: Dedicated
The dedicated strategy cares little for existing structures of the large-scale chemical industry. Instead, it builds entirely new structures with new processes to create new raw materials, through biotechnology, wood or electro chemistry. These products often use biomass or CO2 more efficiently and show properties that are not found in any petrochemical counterpart. However, this would require a large amount of time and resources for building up production capacities, and place the products on the market. Dedicated strategies include replacing petrochemical plastic packaging with paper, cellulose or natural fibre packaging.
Both strategies are necessary to achieve the transformation. While the first is mainly suitable for bulk chemicals, the second could be deployed for small-volume special applications. The first strategy would entail adapting the plants of the large-scale chemical industry, chemical parks, integrated sites and oil refineries, that have been optimised over decades, to the new raw materials and intermediate products of renewable carbon.
This requires considerable investment in structural changes, as well as the development and integration of new technologies (e.g. electrochemistry).
The second scenario is heavily influenced by research and innovation, as it often involves the creation of production routes (e.g. biotechnology) for products with different new, and improved properties. This is especially true for fine chemicals with lower volume, but that allow for higher quality products.
While chemical recycling and large-volume CO2 utilisation are usually more suited to the first pathway, biomass (e.g. for fermentation) is often redestined for the second pathway. The reality, however, is not as black and white as it seems, and one can find any combination between renewable carbon source, process and application that makes sense under certain conditions.
Only Together
What is clear is that this transformation must be worked upon and implemented with high levels of collaboration, all along the value chain: from renewable raw materials via new processes and intermediate products, to final outputs and how to treat them at the end of their lifecycle. From the raw material supplier to the brand manufacturer and trader, the entire value-added chain must be implemented, physically, but also on a political, social and economic level, and supported by marketing. It should be noted that the renewable products will be more expensive. Carbon will never be as cheap as it was in the fossil fuel age, as fossil carbon did not have to bear its external costs. Carbon products will increase in value, meaning that it will be worthwhile to use them for longer time frames; share and re-use them where possible; and to collect and recycle them where not.
Recycling
Developing technologies to share, re-use and recycle products will be essential to keep carbon in the loop. Today, mechanical processes are predominant in recycling. However, these have limitations in terms of what types of waste-streams they can recycle and the
quality of the recyclates. The full potential of recycling can only be unlocked with additional innovative processes such as chemical recycling. In this way, practically all waste fractions, especially mixed ones, can be recycled and turned into high quality feedstock. First, however, large investments need to be made to implement the necessary capacities of chemical recycling plants. With mechanical and chemical recycling, large parts of the carbon (but not all) remains in the cycle. In addition to recycling, other sources of enewable carbon are needed to close gaps in the cycle and minimize the losses. These sources are biomass and direct CO2 use.
Biomass
The biomass breaks down into either primary biomass from fields and forests, or secondary biomass derived from biogenic waste and side streams (e.g. generated by the agriculture and forestry sector, the food,feed and chemicals industries, the production of wood and paper, and private households). Utilising this kind of “organic waste” will be key in transitioning to a biobased circular economy.
The utilisation of biomass makes particular sense wherever functional and complex molecular units of the biomass remain intact after chemical conversion, so they can be used further. For instance, oleochemical, natural rubber and lignin applications qualify in this respect as do numerous novel biobased components such as organic acids and furan based products. Washing, cleaning and care agents, as well as polymers based on these new components, frequently outperform existing products with regards to health and environmental benefits. Additionally, industrial biotechnology may aid in manufacturing complex molecules, using short and gentle processes and made-to measure production organisms. Lignin, for instance, a by-product of wood processing, has been little used to
date, however, in the future it could be used in the production of aromatic compounds and asphalt.
Already today, significant quantities of chemicals – between 10 and 15% depending on the world region – are produced on the basis of biomass. Of course, any further expansion of biomass production must pay strict attention to food security and biodiversity loss. Still, experts see potential for sustainable expansion and, above all, prospective yield, efficiency and storage improvements. Despite sometimes unfavourable public perception, food crops are often a good choice for industrial utilisation. This is due to the fact that they produce very high yields per hectare, and often provide protein-rich by-products, therefore, they make for highly efficient and potentially sustainable land-use solutions. Another option is to use the land and facilities that are currently used for biofuel production, as the demand for liquid fuels is set to dwindle over the next few decades due to the rise of electric cars and hydrogen drives. Advanced biodiesel (HVO) is almost identical to naphtha and ethanol, and canfor PE and PET.
There are also some promising approaches for the use of marine biomass, such as micro and macro algae. These have not yet penetrated mass markets, however, they are used for products such as cosmetics and food supplements. Today, the total volume of marine biomass lags far behind agricultural and forestry systems.
Overall, biomass will not be sufficient to provide enough renewable carbon on its own. Therefore, it is a good thing that biomass is not alone. Which brings us to the third source of renewable carbon: CO2.
Direct Utilisation of CO2
An almost endlessly available source of renewable carbon is carbon dioxide (CO2) and other carbon oxides (e.g. CO) contained in exhaust gases, waste air and the atmosphere. Each of these may be utilised as a raw material for the chemical industry through a number of different technologies.
The field of Carbon Capture and Utilisation offers a wide range of applications, where CO2 can be used as feedstock for chemicals, polymers, fuels, minerals and even proteins. By combining CO2 with green hydrogen, several intermediates and end-products can be produced, such as methane and methanol. In combination with CO2 based formic acid, these can be used as a base feedstock for all kinds of chemicals, polymers and fuels. Synthetic naphtha, for example, can directly replace crude oil naphtha in existing refineries, and can be produced from CO2 and hydrogen through the Fischer-Tropsch reaction. Synthetic naphtha also allows to derive basic chemicals for the production of
higher-grade chemicals and polymers, as well as longchain waxes with high purity levels and value. Some chemicals are by default directly synthesised from CO2, such as urea and other diverse polymers (e.g. polyurethanes and polycarbonates).
In order to make the carbon contained in CO2 reusable, it must be chemically reduced, which requires large amounts of energy, mostly in the form of hydrogen. From an ecological standpoint, this means that only renewable energies or existing process energy can be used for CCU processes to ensure they are sustainable. For this reason, looking ahead, there must be massive, worldwide growth in renewable energies such as solar and wind energy, hydropower and geothermal energy. Based on current trends it is possible to say that this transition is achievable, as many energy systems around the world are already shifting towards renewable options. As shown in the previous chapter, only 0.4 percent of the area of all subtropical deserts would be needed to provide photovoltaic energy for the carbon supply in the depicted 2050 scenario.
The big advantage of CCU technologies over biomass is their high land efficiency and ability to utilize inarable land types like deserts. Disadvantages of CCU include: high investment costs; energy requirements; and the need for construction of new infrastructure. On one hand, the right incentive systems must be created and, on the other, awareness should be fostered that CO2 utilisation, while principally feasible for any application, is especially suitable for the production of bulk chemicals.
According to a Nova press release, there is currently a lot of interest in renewable and green chemistry, bio-based chemistry, CO2 Capture and Utilisation and chemical recycling. Refineries and verbund sites of large-scale chemicals are under considerable pressure; the biofuel industry is looking for new markets in chemistry and start-ups are looking for investors.
Producers of ethanol from biomass or CO2 rich off gases together with plastic producers and refineries are increasingly interested in efficient ways of ethylene production and existing technologies. Producers of HVO advanced biodiesel can also supply bio-naphtha with a few modifications and need to find markets. Newly developed and dedicated chemicals and polymers like PEF need to find their way to the markets, and companies want to know how these will develop in future. Brand owners are not only interested in the immediate polymer procurement intelligence, but also in a secure upstream supply chain for various chemical building blocks. Producers of packaging materials and consumer goods are looking for more sustainable and renewable carbon-based solutions for their products with the same or better properties. Companies with potential feedstocks like bio-wastes or CO2-rich
off-gas streams are looking for technologies to valorise these and need to know about available technologies, costs and potential markets.
Conclusion
Since the beginning of the industrial revolution, humanity has almost exclusively relied on cheap virgin fossil carbon sources such as coal and crude oil for its development. Today, for the first time, we can now decouple the production of chemicals and derived materials
from the use of virgin fossil carbon. All of today‘s chemicals and derived products can be made with renewable carbon from biomass, captured CO2 or recy-able for the transformation from fossil to renewable carbon for the entire industry. The renewable carbon family is the only pathway to a truly sustainable future for the chemical industry.
Renewable carbon reports available from Nova:
1. The need for bio-based carbon in a sustainable future – http://nova-institute.eu/press/?id=271
2. How to meet the global need for carbon as a feedstock in the chemical and derived materials sector in the future? – http://nova-institute.eu/press/?id=259
3. Minimising investment risks in renewable chemistry – http://nova-institute.eu/press/?id=276
4. Carbon economy – http://renewable-carbon.eu/publications/
Some examples of carbon dioxide utilisation which won awards at the 9th conference on CO2 based fuels and chemicals (Excerpted from a Nova press release)
First Place : Carbon Recycling International (Iceland): Emissions-to- Liquids Technology
Carbon Recycling International (CRI) has been developing its Emissions-to-Liquids (ETL) technology since 2006. CRI’s first industrial demonstration plant was commissioned in Iceland in 2012. It has a capacity of 4,000 tonnes methanol per year. ETL converts CO2 and hydrogen into methanol in a one-step reaction. In 2020 the company moved from industrial demonstration scale to full commercial scale with a project in Anyang, Henan province, China. The plant, which will have a capacity of 110,000 tonnes methanol per year, will be commissioned in 2021. This represents an important step for CO2 hydrogenation projects worldwide. The CO2-based methanol is used for gasoline blending, biodiesel esterification, fuel cell operation, waste-water denitrification and chemicals production. More information: http://www.carbonrecycling.is
Second Place: LanzaTech (USA): CarbonSmart Ethanol
The CarbonSmart Ethanol technology allows to capture carbon dioxide and to creating value from waste; effectively turning pollution into products. LanzaTech uses microorganisms to make ethanol from the CO2 and CO emissions of industrial processes like steel, syngas from municipal solid waste, waste wood or waste plastics. Ethanol is a chemical building block that can be used to produce a broad range of everyday products from household cleaning products, plastic shampoo bottles, furniture and toys, to fine fragrances and sporting goods. With a commercial plant in China that has produced over 20,000 tonnes of ethanol and mitigated over 100,000 tonnes of CO2 since 2018, the technology is rapidly gaining traction in the market. The steel company ArcelorMittal (Luxembourg) licensed LanzaTech’s technology and is building the first European plant to convert steel off-gases into CarbonSmartTM Ethanol in Ghent (Belgium). The plant is estimated to have a capacity of 62,000 tonnes of ethanol per year and to mitigate over 350,000 tons of CO2 per year.
More information: http://www.lanzatech.com
Third Place: Covestro (Germany): Washing with CO2-Technology – Surfactants based on CO2
CO2 instead of petroleum – Covestro is pursuing new avenues for turning the waste gas into a useful raw material. The goal is to use CO2 as resource in as many applications as possible. And the next scope is here to come: Surfactants based on CO2. First application
tests have shown that these CO2-based surfactants can be used in a standard detergent formulation. The product development process is still in progress and the boundary conditions are already set: less fossil- based, less global warming potential, readily biodegradable and comparable washing performance – a new class of more sustainable surfactants for the everyday businesses.
More information: http://www.covestro.de