Abstract
Ever since concerns of global warming, climate change due to green house gases emissions have gripped the world, industries have been looking at ways and means to decarbonize, as carbon and its conversion to CO2 seems to be the major culprit. The prospects of transiting to a hydrogen economy is being widely discussed, provided the hydrogen is produced through renewable energy – in which case it is termed as green hydrogen. Since then colour wise, multiple colour nomenclatures of hydrogen have come up like grey, blue, even white, yellow, turquoise etc. This article explains those color coding of hydrogen and what kinds of hydrogen are economically feasible and viable in the current global economic scenario as well as technologically.
Introduction
In an effort to achieve global net zero emissions, clients worldwide have jumped the bandwagon and are considering Hydrogen as the fuel of the future. Hydrogen as fuel will contribute in a big way in leading the decarbonization charge. The variable and non-dispatchable nature of wind and solar energy is hindering them to be the immediate solution to this energy issue. Interestingly, the industry players use different color codes for hydrogen to distinguish between different technologies used to produce hydrogen gas.
Green hydrogen in simple terms is hydrogen that is produced via electrolysis. Electrolyzers use an electrochemical reaction to split water into hydrogen and oxygen. It is extremely environment friendly and effective as zero carbon dioxide is emitted during this process but may be limited by capacity.
Grey hydrogen is predominantly produced from natural gas using a process called steam reforming. It is the primary source of hydrogen in all refineries and petrochemical complexes. The CO2 thus generated, if removed via subsequent Carbon Capture (CC) techniques, can then term the hydrogen as blue. This method of blue hydrogen production doesn’t fully remove the greenhouse gases but with the use of carbon capture techniques, climate neutrality can be achieved. Existing steam reforming units are also planning to implement Carbon Capture techniques to improve their carbon footprint. This provides an opportunity for EPC players to support such endeavors.
Brown hydrogen is the one produced via the coal gasification techniques and the greenhouse gases emanating out of this process are still a major cause of concern. Brown and Grey hydrogen generated from fossil fuels contribute almost the entire amount of the total hydrogen produced worldwide, and any major EPC player can tap into this market to support clients and provide integrated solutions to eliminate or reduce the emission of greenhouse gases.
Another interesting color coding of H2 is Turquoise. This H2 is a byproduct of methane pyrolysis which generates carbon or coke instead of CO2. But typically,it is the hydrogen of least choice.
Other color definitions provided to hydrogen include yellow, as produced via electrolysis using solar power; white, as produced via naturally occurring geological hydrogen found in underground deposits and created through fracking; or even pink or purple, generated through electrolysis powered by nuclear energy. But there are no strategies to exploit them at present.
The big question is which color hydrogen is the fuel of the future. Studies suggest that most clients worldwide are opting for blue hydrogen. Large scale implementation of carbon capture techniques in existing facilities provide the opportunity for global EPC players to collaborate with clients worldwide.
Green Hydrogen
Green hydrogen as the name suggests is the hydrogen which is most environment friendly and with the minimum carbon footprint. Green hydrogen is produced by splitting water using electricity generated from low carbon sources. If the electricity comes from renewable sources such as wind, solar or hydro, then the hydrogen is effectively green.This is the cleanest form of hydrogen with zero emissions and results in a cleaner fuel. This is the cleanest form of hydrogen with zero emissions and results in a cleaner fuel.
Energy agencies worldwide suggest that this method of obtaining green hydrogen would save millions of tonnes of CO2 that are emitted annually when hydrogen is produced using fossil fuels. However, this hydrogen production has certain pros and cons associated with its generation. It’s a very usable product with respect to mobility aspects and is a highly sustainable fuel since it does not lead to emit polluting gases neither during combustion and nor during production. But the aspect about green production which makes it a fuel of lesser liking is that it requires more energy than other fuels and add to that the 24/7 availability. The other feature that goes against the choice of using green hydrogen is the economics. The costs involved to generate green hydrogen is highly skewed at present, almost thrice to the hydrogen generated via the conventional route. In a report published by an international agency a few years ago suggests that the cost of green hydrogen at $3 to $7.50 per kilo, compared to $0.90 to $3.20 for production using steam methane reformation. Important aspect to note is that reducing the cost of electrolyzers will be critical to reducing the price of green hydrogen, but that will take time and scale. Electrolyzer costs could fall by half by 2040, from around $840 per kilowatt of capacity today. Many European nations have jumped into the bandwagon and unveiled a national hydrogen strategy to develop green hydrogen in a big way and scale up for future.
Grey Hydrogen
Steam methane reforming (SMR) is the most common technique for producing grey hydrogen. Several types of fossil fuels can serve as an input in this process, with natural gas being the most common. This process yields carbon in addition to hydrogen. If this carbon is released into the atmosphere, the hydrogen produced is considered ‘grey hydrogen’. In the present global economic conditions, the grey hydrogen has one of the lowest overall fixed and variable costs of production and requires a smaller footprint. Feedstock, typically natural gas or other varied sources such as LPG or naphtha, may also be used as feed for the grey hydrogen generation process.
The production costs of grey hydrogen are estimated to be between $1/kg and $1.60/kg. This is currently the cheapest method to produce hydrogen. Currently, the CO2 resulting from the production of fuel-based hydrogen is mainly emitted into the atmosphere. Only a small part is captured and used in the production process of some types of fertilizers or sold commercially. Since the market for the commercial use of fossil-based CO2 is limited and will remain so, CO2 needs to be captured and stored in order to convert the grey hydrogen production to blue. The trade-off for grey versus blue hydrogen production is between CO2 emission costs and CCS (Carbon Capture and Sequestering) costs.
Blue Hydrogen
Blue hydrogen is the hydrogen generated using fossil fuels with carbon capture and storage. Blue hydrogen is produced when natural gas is split into hydrogen and CO2 predominantly by Steam Methane Reforming (SMR), but the CO2 is captured and then stored. As the greenhouse gases are captured, this mitigates the environmental impact on the planet. Blue hydrogen is often recognized as a low-carbon fuel for generating electricity and storing energy, powering cars, trucks and trains and heating buildings.
According to the International Energy Agency, 96% of hydrogen produced worldwide is made using fossil fuels – coal, oil and natural gas – in a process known as reforming. This involves combining fossil fuels with steam, and heating them to around 800°C. Eventually, you get carbon dioxide (CO2) and hydrogen. These gases are then separated. The CO2 is mostly emitted to the atmosphere where it contributes to global heating, and the hydrogen is extracted and used in everything from car engines to boilers, releasing water vapor.
The process of making blue hydrogen also requires a lot of energy. For every unit of heat in the natural gas at the start of the process, only 70-75% of that potential heat remains in the hydrogen product. In other words, if the hydrogen is used to heat a building, you would need to use at least 25% more natural gas to make blue hydrogen than if it was used directly for heat. And as reported by an international agency, methane – the primary component of natural gas and a byproduct of using it to produce blue hydrogen – is a much more potent global warming gas than CO2 over shorter timescales. On a 100-year basis, methane has a global warming potential 28-36 times greater than CO2, so one molecule of methane in the atmosphere has the same effect as around 30 molecules of CO2.
Absorption with aqueous amine solutions e.g., monoethanolamine (MEA), diethanolamine (DEA), etc. is conventionally used to capture CO2 at large scale. The low solvent cost and good trapping effect make it the most popular and developed carbon capture technology. This strategy has a wide range of applications and can be applied to capture CO2 from streams with low concentrations. However, it presents several disadvantages such as high-energy consumption required for regeneration, degradation in the presence of oxygenated compounds, loss of amines by evaporation, and corrosion. Alternatively, solid adsorbents are becoming popular aimed at improving the shortcomings of amine solutions. As such, the use of adsorbents has been proposed as an alternative for CO2 capture. Adsorbents can exhibit high affinity for selective CO2 adsorption under flue gas conditions. Solid adsorbents with amine functionality are promising materials for the capture of CO2 because of its lower heat capacity compared to liquid amine solutions. Stable performance for CO2 capture under humid conditions and regenerability of the adsorbent are critical to the realization of practical CO2 adsorbents.
There is also the option of the location of the carbon capture block in the hydrogen generation process flow schemes. There are two main locations from where the CO2 may be captured. (1) The high-pressure syngas stream upstream of the PSA (pre-PSA syngas); or (2) The reformer furnace flue gas stream. Each process has its own strengths and limitations, and hence the actual implementation of the carbon capture scheme would be determined by the existing SMR process flow scheme. The additional long-term challenge of carbon storage and disposal also would need to be addressed whenever CCS is applied.
Conclusion
In the present operating scenarios of refineries and petrochemical complexes, almost the entire amount of the hydrogen is produced via the steam methane reforming method. Although it poses a threat to the climate change issues with its high carbon footprint, the current economics is not allowing scale-up of green hydrogen and become an immediate replacement. Green hydrogen may seem the long-term solution for this issue, but for the immediate future, revamping the existing hydrogen plants producing grey hydrogen to generate blue hydrogen seems to be the potential path forward. Carbon capture techniques have been in place for a pretty long time, and with its established technology, it would provide a solution to mitigate this challenge. This allows all EPC players and clients to collaborate and select the CCS technology to be implemented in their facility for a greener future ahead for all of us.
Author :
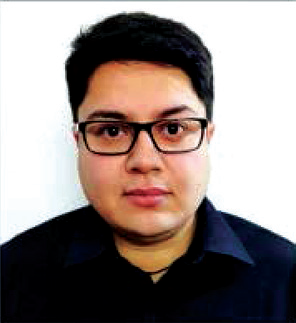
Fluor Daniel India Pvt Ltd, New Delhi has more
than 12 years of experience in petroleum refining,
petrochemicals & fertilizer projects. He holds
a Master’s degree in Chemical Engineering from
the Indian Institute of Technology, Kharagpur.
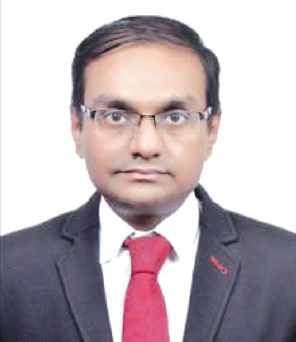
(The conclusions presented in this article are solely those of the authors and cannot be ascribed to Fluor Corporation and/or any of its subsidiaries.)